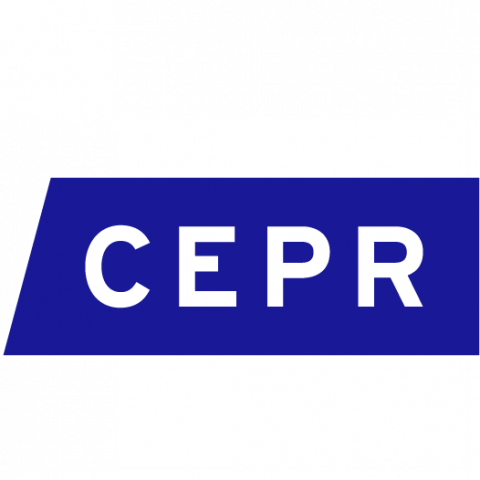
A large-scale study establishes what is believed to be the first causal link between in-utero exposure to microplastics and low birthweights.
An estimated 8300 million metric tons of virgin plastics have been produced worldwide, with most of these materials (79%) accumulating in landfills or the natural environment (Geyer et al. 2017). These materials then break down into ever smaller pieces, creating microplastics that can stay in the environment – soil, water and air – indefinitely (Rillig 2012).
Microplastics (and even tinier nanoplastics) have been found practically everywhere, in the food we eat, water we drink, the air we breathe. Unsurprisingly, they have also been detected in the human body.
As concerns grow over the ubiquitous presence of microplastics, researchers are seeking to understand whether exposure to these particles harms human health. Assessing the direct impacts of such exposure is a difficult task. Studies thus far have largely relied on small-scale, cross-sectional samples to examine potential impacts on, for example, fetal development (Amereh at al. 2022), respiratory systems (Baeza-Martinez et al. 2022) and inflammatory bowel diseases (Yan et al. 2022).
Using satellite data and birth records around the world to measure the impact of microplastics
Our recent work takes an entirely different approach. We examine the potential harm from plastics exposure on a very global scale. Our study examines links between microplastics exposure and the incidence of low birthweight, a primary measure of infant health that has been linked to environmental stressors (Currie and Almond 2011). It relies on data from more than three million births between 2017 and 2018 across coastal areas of the world, spanning four continents and 15 countries, and including countries of different income levels.
We assessed whether low birthweights (below 2,500 grams) were more prevalent when expectant mothers had been exposed to conditions that generated higher levels of microplastics particles in the coastal air.
We were able to examine this relationship by using data from satellites, which show variations in the presence of microplastics in the world’s oceans by detecting the wind-dampening effects that microplastics have on the ocean surface (Evans and Ruf 2021). We created models to incorporate this data on the presence of marine microplastics to calculate the times for ocean and wind currents to carry various concentrations of microplastics toward the shore and to account for prevailing evaporation rates. Using this combination – the random presence of microplastics in the open sea, variability in ocean and wind currents, and measures of air temperatures that influence evaporation rates – we were able to determine when conditions were ripe for microplastics to be aerosolised from ocean waters into the coastal air and, thus, when people in coastal areas were more exposed to airborne plastics pollution.
Figure 1: Coastal areas of the world exposed to microplastics
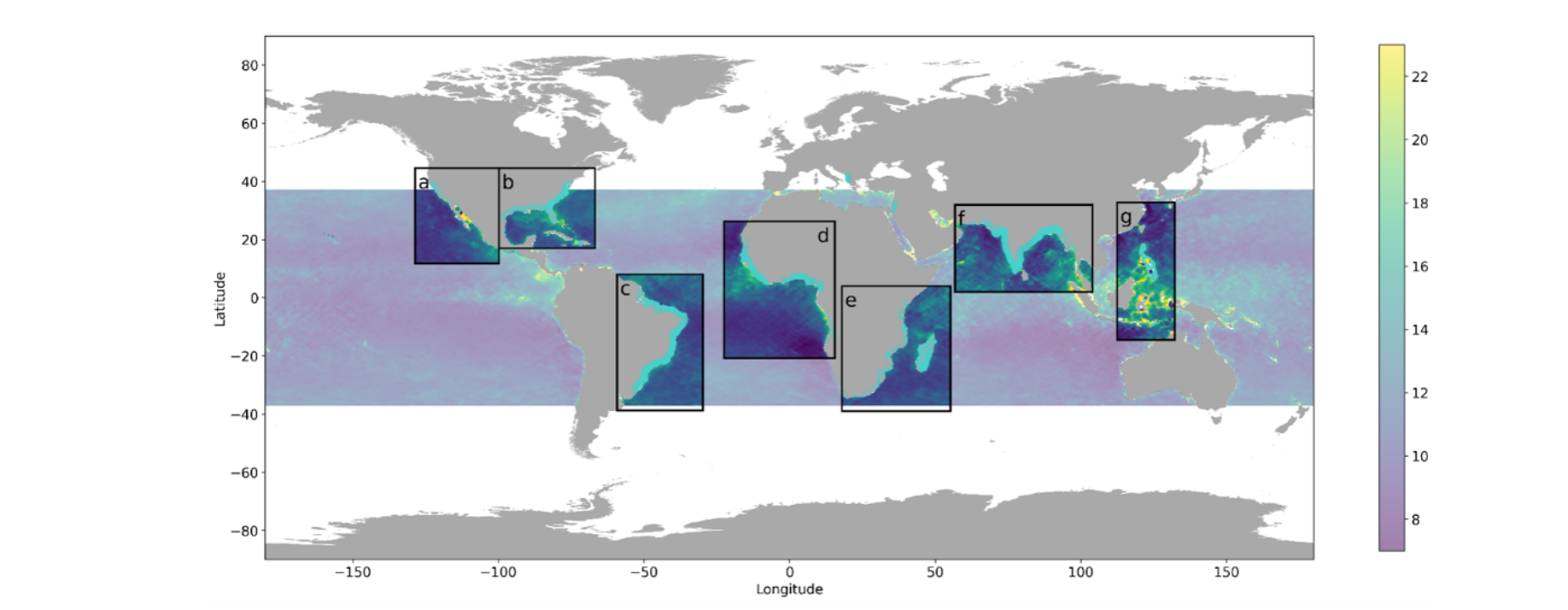
Notes: Distribution of microplastic concentration from the remote-sensing measurement.
Our work is guided by research in a wide array of fields, including atmospheric, oceanic, biological, medical, and environmental toxicology fields. It also builds on work that has generated new understanding about how microplastics can move from water to air, for example, by bubbles that burst in bodies of water (Harb et al. 2023) and by raindrops falling (Lehmann et al. 2021), and how these particles can travel, moved by sea spray, sea currents and wind (Allen et al. 2020, Caracci et al. 2023).
Establishing a causal link between airborne microplastic exposure and low birthweights
Our work establishes what we believe to be a first connection between in-utero exposure to microplastics and harm to infant health, as indicated by lower birthweights. Potential endogeneity exists as microplastics may correlate with other health-affecting factors. We develop an ocean transport model that leverages day-to-day changes in ocean current to predict how microplastics from the open sea can be expected to reach coastal locations.
Figure 2: Example of how changes in ocean currents can predict how microplastics reach coastal locations
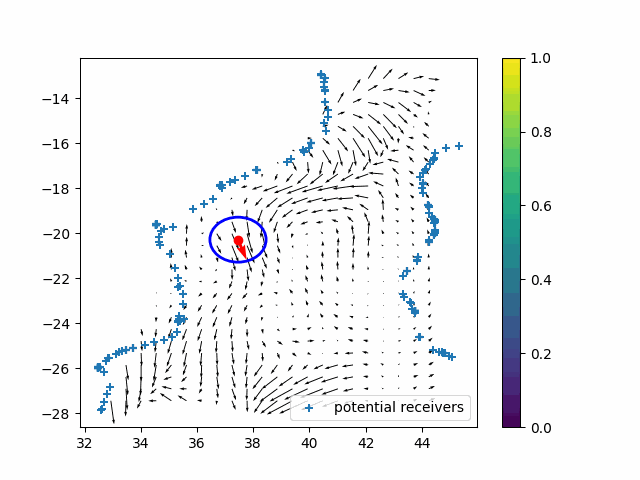
Notes: An illustration of the transport modelling that tracks coastal variation in microplastic exposure due to microplastic presence from sea surface afar.
Our findings show that exposure to marine microplastics, particularly during the second and third trimesters, leads to a significant increase in the likelihood of low birthweights. A doubling of exposure increases the risk of low birthweight by 0.37 per 1,000 births, suggesting that more than 205,000 cases per year globally can be attributed to microplastic exposure. The rates ranged from approximately 1.2% in the US to more than 8% in developing countries in Africa and South Asia.
Figure 3: The effect of exposure to marine microplastics during the trimesters of pregnancy
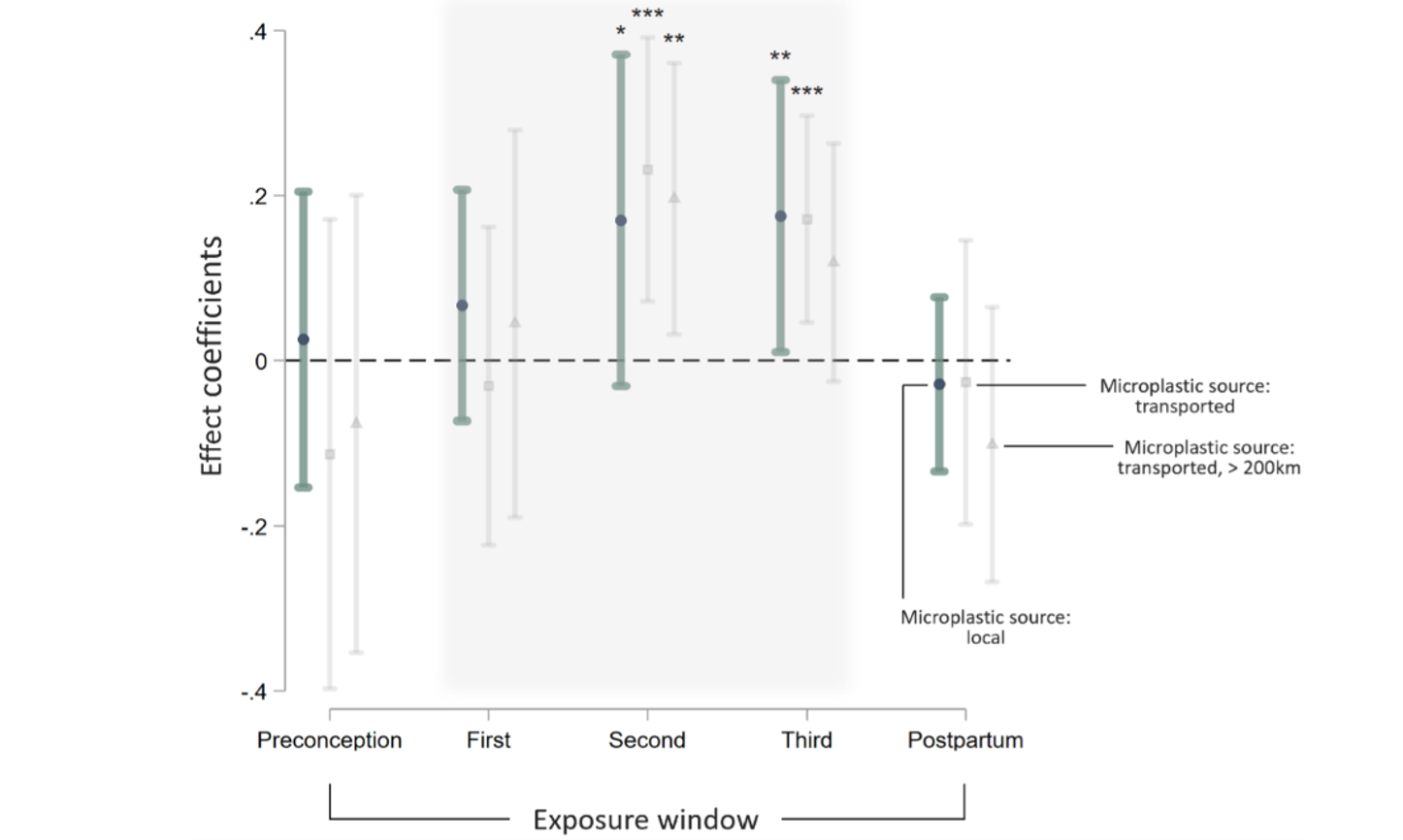
Notes: This figure shows coefficient estimates from three separate regressions looking at the impact of in-utero microplastic exposure on incidents of low birth weight. The regressions use different microplastic measurement (circles for local microplastic source, squares for transported source, and triangles for transported source with a 200km buffer) but are otherwise with identical specification. “Preconception” corresponds to microplastic exposure during the 3-month period prior to conception, and “Postpartum” corresponds to exposure during the 3-month periods after birth. *: p < 0.10; **: p < 0.05; ***: p < 0.01.
How do microplastics affect infant health?
Our data reveals a strong correlation between microplastic levels and the concentration of coastal aerosol particulate pollution, with this relationship intensifying under higher evaporation rates in coastal areas. We estimate that this pathway explains approximately half of the total impact of microplastics on low birth weight, indicating that aerosolisation is a primary mechanism through which microplastics affects infant health.
How to tackle the serious risks of microplastic exposure
Our findings corroborate decades of research across multiple fields warning about the potential risks of microplastic exposure. At the same time, our findings reveal that research is needed to understand how exposure of the developing fetus to microplastics may impact short- and long-term health outcomes.
The results also suggest that microplastics are a new and pervasive source of airborne particulate pollution. This raises important questions about the broader health impacts on all ages. Extensive scientific evidence has long established that fine particulate matter in the air has adverse impacts on human health, particularly for respiratory and cardiovascular functions (e.g. Landrigan et al. 2018). Such findings underpin regulations of particulate matter that have been implemented by many governments. The World Health Organization global air quality guidelines are based on an increasingly strong body of evidence showing that air pollution harms various aspects of health, and at even lower concentrations than once had been believed. Moreover, prior research has established a causal link between airborne particle pollution and adverse infant health outcomes (e.g. Jayachandran 2009, Currie and Schwandt 2016, Alexander and Schwandt 2022).
References
Allen, S, D Allen, K Moss, G Le Roux, V R Phoenix, and J E Sonke (2020), “Examination of the ocean as a source for atmospheric microplastics,” PloS ONE, 15(5): e0232746.
Amereh, F, N Amjadi, A Mohseni-Bandpei, S Isazadeh, Y Mehrabi, A Eslami, Z Naeiji, and M Rafiee (2022), “Placental plastics in young women from general population correlate with reduced foetal growth in IUGR pregnancies,” Environmental Pollution, 314: 120174.
Baeza-Martínez, C, S Olmos, M González-Pleiter, J López-Castellanos, E García-Pachón, M Masiá-Canuto, L Hernández-Blasco, and J Bayo (2022), “First evidence of microplastics isolated in European citizens’ lower airway,” Journal of Hazardous Materials, 438: 129439.
Bryan, G, S Chowdhury, and A M Mobarak (2014), “Underinvestment in a profitable technology: The case of seasonal migration in Bangladesh,” Econometrica, 82(5): 1671–1748.
Caracci, E, A Vega-Herrera, J Dachs, N Berrojalbiz, G Buonanno, E Abad, M Llorca, T Moreno, and M Farré (2023), “Micro (nano) plastics in the atmosphere of the Atlantic Ocean,” Journal of Hazardous Materials, 450: 131036.
Chetty, R, N Hendren, and L F Katz (2016), “The effects of exposure to better neighborhoods on children: New evidence from the moving to opportunity experiment,” American Economic Review, 106(4): 855–902.
Chetty, R, and N Hendren (2018), “The impacts of neighborhoods on intergenerational mobility I: Childhood exposure effects,” The Quarterly Journal of Economics, 133(3): 1107–1162.
Currie, J, and D Almond (2011), “Human capital development before age five,” in Handbook of Labor Economics, Vol. 4, ed. by D Card and O Ashenfelter, pp. 1315–1486, Elsevier.
Currie, J, and H Schwandt (2016), “The 9/11 dust cloud and pregnancy outcomes: A reconsideration,” Journal of Human Resources, 51(4): 805–831.
Evans, M C, and C S Ruf (2021), “Toward the detection and imaging of ocean microplastics with a spaceborne radar,” IEEE Transactions on Geoscience and Remote Sensing, 60: 1–9.
Fan, X, H Shao, M Sakamoto, K Kuga, L Lan, D P Wyon, K Ito, M P Bivolarova, C Liao, and P Wargocki (2022), “The effects of ventilation and temperature on sleep quality and next-day work performance: Pilot measurements in a climate chamber,” Building and Environment, 209.
Harb, C, N Pokhrel, and H Foroutan (2023), “Quantification of the emission of atmospheric microplastics and nanoplastics via sea spray,” Environmental Science & Technology Letters, 10(6): 513–519.
Jomo, K (2017), “The new economic policy and interethnic relations in Malaysia,” in Global Minority Rights, pp. 239–266, Routledge.
Kabeer, N (1999), “Resources, agency, achievements: Reflections on the measurement of women’s empowerment,” Development and Change, 30(3): 435–464.
Kok, C C, and G J Lim (2024), “Ethnic proximity and politics: Evidence from colonial resettlement in Malaysia,” Available at SSRN 5008727.
Landrigan, P J, R Fuller, N JR Acosta, O Adeyi, R Arnold, A Bibi Baldé, R Bertollini, et al. (2018), “The Lancet Commission on pollution and health,” The Lancet, 391(10119): 462–512.
Lehmann, M, L M Oehlschlägel, F P Häusl, A Held, and S Gekle (2021), “Ejection of marine microplastics by raindrops: A computational and experimental study,” Microplastics and Nanoplastics, 1: 1–19.
Pavanello, F, E De Cian, M Davide, M Mistry, T Cruz, P Bezerra, D Jagu, S Renner, R Schaeffer, and A F P Lucena (2021), “Air-conditioning and the adaptation cooling deficit in emerging economies,” Nature Communications, 12(1): 6460.
Prata, J C (2019), “Airborne microplastics: Consequences to human health?” Environmental Pollution, 24(2-19): 115–126.
Rillig, M C (2012), “Microplastics in terrestrial ecosystems and the soil?” Environmental Sciences & Technology, 46: 6453–6454.
Togo, F, S Aizawa, J I Arai, S Yoshikawa, T Ishiwata, R J Shephard, and Y Aoyagi (2007), “Influence on human sleep patterns of lowering and delaying the minimum core body temperature by slow changes in the thermal environment,” Sleep, 30(6).
Van Dongen, H P A, G Maislin, J M Mullington, and D F Dinges (2003), “The cumulative cost of additional wakefulness: Dose-response effects on neurobehavioral functions and sleep physiology from chronic sleep restriction and total sleep deprivation,” Sleep, 26(2).
Yan, Z, Y Liu, T Zhang, F Zhang, H Ren, and Y Zhang (2021), “Analysis of microplastics in human feces reveals a correlation between fecal microplastics and inflammatory bowel disease status,” Environmental Science & Technology, 56(1): 414.